COVID-19 Transmission Assessment Report

Looking for this report as a PDF?
Download the COVID-19 Transmission Assessment Report
High Velocity Nasal Insufflation (HVNI) Therapy Application in Management of COVID-19
Leonard, S. BSME1, Volakis, L.I., MS PhD1, DeBellis, R., PharmD FCCP1, Kahlon, A., MD1,
Mayar, S., MSc RRT1, Dungan II, G.C., MPhil (Medicine)1,2,3
- Department of Science & Innovation, Vapotherm, Inc. Exeter, NH USA
- Education and Human Services, Canisius College, Buffalo, NY USA
- CIRUS, Sydney, NSW Australia
Executive Summary
COVID-19 is a viral pneumonia which emerged in 2019 and reached Pandemic status by March 2020. The disease presents with a range of symptoms, up to profound hypoxemic respiratory failure. Management recommendations around the world call for the use of High Flow delivery of high flow oxygen – such as High Velocity Nasal Insufflation (HVNI). A caution with HVNI arose because of a concern for generation droplets and aerosols created or propelled by the therapy. Literature review shows 1) all respiratory therapy has potential to create aerosols, and 2) High Flow therapies, when properly applied, are low risk compared to NIPPV and airway management. Personal Protective Equipment (PPE) is essential with any respiratory therapy for COVID-19 patients. A surgical mask over the face during HVNI has been posited from experiences in China. A Computational Fluid Dynamic simulation shows that for HVNI therapy with a surgical mask in place over the patient’s face the percentage of particles which remain airborne or deposit on a surface further than the immediate area of the bed is similar to low flow oxygen with a mask or a patient breathing with no therapy wearing a mask. Leaks are greater with HVNI and can be associated with a small percentage of particle mass escape from the mask. Minimizing the mask leak is recommended to further reduce risk to the care giver.
OBJECTIVE
HVNI is a potential therapeutic adjunct in the management of acute respiratory failure associated with COVID-19 viral pneumonia. The Risk versus Benefit ratio of any application of any respiratory therapy providing ventilatory and oxygenation support to such patients must be considered, along with considerations of resource management. Clinical efficacy and likelihood of success, ease of use, simplicity of interface, and known or suspected risks (including inadvertent transmission) must enter into that clinical decision. This paper will help provide a factual framework to assist in that clinical and practical analysis. Ultimately, the clinician managing the patient is responsible for maximizing the successful management of the patient, the prudent use of clinical resources, and the mitigation of risk to the patient, staff, and environment. This document is the result of both a literature review and in silico modeling. A literature review of salient background information was collected using a directed search beginning with key known guidelines and clinical papers, augmented with NCBI PubMed and Google Scholar. Key areas of investigation are individually addressed in the body of the paper. This literature review, compilation and summarization led to a conclusive narrative. This review was also informed by in silico testing, specifically designed to evaluate important aspects of potential environmental contamination associated with aerosol generation attendant with HVNI, including the geometric dynamics of such potential environmental contamination whilst employing the efficacy of a prudent and resource/procedurally disintensive mitigation (specifically a simple surgical mask over the HVNI interface whilst on the patient’s face).
CONCLUSIONS
COVID-19 can present with respiratory failure and Acute Respiratory Distress Syndrome (ARDS) having progressed from pneumonia. This expression can include acute respiratory failure, typically Type I (hypoxic). Such failure may be amenable to management using HVNI therapy provided the patient is spontaneously breathing, and the use of HVNI is consistent with international guidelines. HVNI is associated with specific clinical and environmental advantages over other non-invasive ventilatory support. HVNI has been shown to be an effective tool to treat all cause respiratory failure in adults. HVNI is an open-interface high flow oxygen delivery and would be included in the recommendation for COVID-19 management guidelines. Mitigation of the potential for nosocomial transmission associated with Aerosol Generating Procedures (AGP) may be achieved using a properly fitting surgical facemask over the patient’s HVNI interface (cannula).
INTRODUCTION / BACKGROUND
A recent Lancet Respiratory Medicine Correspondence by Cheung et al., concluded that: (1) all Aerosol Generating Procedures (AGPs), including High Flow Nasal Cannula (HFNC), are high risk for COVID-19 infection transmission; and (2) due to non-universal interfaces across multiple device technologies that HFNC and Non-Invasive Ventilation (NIV and more specifically Non-invasive Positive Pressure Ventilation [NIPPV]), with a potential for poor application to the patient, is not recommended for use without an isolation room [1]. This raises the question of the actual risk of aerosol-borne vectors for nosocomial transmission of the SARS-CoV-2 virus, in the context of existing literature suggesting safe operation. High Flow therapy employs high volumetric flow rates of heated humified air/oxygen mixtures to provide oxygen. HFNC, High Flow Oxygen (HFO or HFNO) and High Velocity Nasal Insufflation (HVNI) all act with an open interface to deliver oxygen to the patient. Collectively, in regard to the ability to deliver oxygen to the nose of the patient, these are similar, however, HVNI differs in that it acts to also provide non-invasive ventilatory support to spontaneously breathing patients.
METHODS
A literature review of salient background information was collected using directed search beginning with key known guidelines and clinical papers, augmented with NCBI PubMed and Google Scholar and key journal searches. Key areas of investigation are individually addressed in the body of the paper. This literature review, compilation and summarization led to a conclusive narrative. This review was also informed by in silico testing, specifically designed to evaluate important aspects of potential environmental contamination associated with aerosol generation attendant with HVNI, including the geometric dynamics of such potential environmental contamination whilst employing prudent and resource/procedurally disintensive mitigation (surgical mask over the HVNI interface whilst on the patient’s face).
Coronavirus Disease – COVID-19
Disease Description
The COVID-19 disease constitutes a serious pandemic with viral pneumonia as the most frequent serious manifestation. It is caused by SARS-CoV-2, a betacoronavirus in the same subgenus as several bat coronaviruses, including the severe acute respiratory syndrome virus (SARS-CoV). The SARS-CoV-2 virus shares 79.6% sequence identity to SARS-CoV and uses the same receptor, angiotensin converting enzyme II (ACE2) to enter the cell. [6]
From the Chinese Center for Disease Control and Prevention that at the time of writing, included approximately 44,500 confirmed COVID-19 cases, 81% of the patients presented with no or mild pneumonia7. Severe disease such as patients presenting with dyspnea, hypoxia, or >50% lung involvement on imaging within 24-48 hours accounted for 14% of the infected. 5% accounted for patients reported to be in critical condition such as respiratory failure, shock, or multiorgan dysfunction (MODS). The overall, case fatality was reported at that time to be 2.3% with no deaths in the noncritical cases. The World Health Organization (WHO) reported “Asymptomatic infection has been reported, but the majority of the relatively rare cases who are asymptomatic on the date of identification/report went on to develop disease. The proportion of truly asymptomatic infections is unclear but appears to be relatively rare and does not appear to be a major driver of transmission.” [8] The Center for Systems Science and Engineering at Johns Hopkins reported 8.244 deaths out of a confirmed positive population of 204,264 (accessed 18 Mar 2020). [9]
According to the WHO, COVID-19 is transmitted via respiratory droplets and fomites during close unprotected contact between the infector and infectee. [8] Airborne spread has not been reported for COVID-19 as of this writing and it is not believed to be a major driver of transmission based on current evidence. Fecal shedding has been demonstrated from some patients, and viable virus has been identified in a limited number of reports, but the fecal-oral route does not appear to be a driver of COVID-19 transmission.
Clinical Presentation / Course
COVID-19 disease presentation can range from asymptomatic to severe pneumonia and death. Based on the 55,924 laboratory confirmed cases, typical signs and symptoms include: fever (87.9%), dry cough (67.7%), fatigue (38.1%), sputum production (33.4%), dyspnea (18.6%), sore throat (13.9%), headache (13.6%), myalgia (14.8%), chills (11.4%), nausea or vomiting (5%), nasal congestion (4.8%), diarrhea (3.7%). [8] COVID-19 patients generally develop signs and symptoms, including mild respiratory symptoms and fever, on an average of 5-6 days after infection (mean incubation period 5-6 days, range 1-14 days). [8] Majority of patients with COVID-19 virus have mild to moderate disease and recover (approximately 80% of laboratory confirmed patients). Moderate disease presentation includes non-pneumonia and pneumonia cases. 13.8% have severe disease (dyspnea, respiratory frequency ≥30 breaths/minute, blood oxygen saturation ≤93%, PaO2/FiO2 ratio <300 mmHg, and infiltrates consuming 50% of the lung field within 24-48 hours) and 6.1% are critical (respiratory failure, septic shock, and/or multiple organ dysfunction/failure).” [8] Mortality increases with age and presence of comorbidities, including chronic medical conditions (13.2% for those with cardiovascular disease, 9.2% for diabetes, 8.4% for hypertension, 8.0% for chronic respiratory disease, and 7.6% for cancer). [8]
COVID-19 has been associated with substantial burden on the ability of healthcare systems to provide necessary support. In particular, the availability of proper facilities (e.g., Intensive Care Units, Infectious Disease Areas/isolation and negative pressure rooms) and equipment (including ventilatory support and oxygen delivery) has become a problem in high density COVID-19 presentation. [10]
High Velocity Nasal Insufflation Therapy
HVNI Mechanism of Action
High Velocity Nasal Insufflation (HVNI) shares characteristics with High Flow Nasal Cannula (HFNC) therapy, in that both deliver heated humidified gas through an open nasal interface. In that, both are able to deliver oxygen-rich gas at supraphysiologic flows, allowing a more precise FiO2 delivery in management of hypoxic respiratory failure. HVNI differs from HFNC in terms of ventilation support for spontaneously breathing patients. The FDA has granted a new product code to the Precision Flow Hi-VNI system, and allowed a claim including providing ventilatory support for spontaneously breathing patients. The fundamental mechanistic difference between HFNC and HVNI is in the velocity of the gas flow delivered into the nose. The high velocity high flow facilitate washout of end-expiratory CO2 from extra-thoracic dead space between breaths as well as during the expiratory phase of ventilation. High velocity gas forms complex vortices facilitating rapid flush of the deadspace, a feature particularly important for tachypneic patients. [11] [12]
Other mechanisms of action for HVNI include the provision of warm and humidified gas to conducting airways to improve conductance, pulmonary compliance and maintenance of mucociliary function. The provision of adequately warmed and humidified gas reduces metabolic workload associated with breathing and the instillation of high velocity gas at high flow rates that is transmitted to high velocity provides modest distending pressure during exhalation. [13]
High Flow therapies (HFNC and HVNI) both provide oxygenation support to patients at sufficient flows to permit reliable high FiO2 delivery. Both have been shown to effectively manage hypoxic respiratory failure in adult patients presenting in the emergency department. The ability to flush is further facilitated by the fluid mechanics of the high velocity gas-stream delivery afforded by the HVNI interface. [12] This principle allows therapeutic management at lower volumetric flow rates. Doshi et al., showed HVNI as non-inferior to NiPPV in management of adults presenting in the ED with undifferentiated respiratory failure, employing a starting volumetric flow rate of 35L∙min-1, and titrating to a mean flow of 30 ± 6 L∙min-1. [14] In a study of hypoxemic respiratory failure patients presenting in the ED, similar comparability was demonstrated with a HFNC system using lower-velocity interface, but at a mean flow rate of 48 ± 11 L∙min-1 (this is important as higher mass flow may also facilitate greater transport of aerosolized particles), and at a higher proportion of failure requiring intubation. [15] Both studies demonstrated adequate oxygenation of the patients.
High Flow therapy in general has demonstrated broad capability to manage acute respiratory failure. In a meta-analysis HFNC was shown to provide superior outcomes regarding endotracheal intubation as compared to conventional oxygen therapy, and comparable rates of intubation compared to NiPPV. [16]
Standards/Recommendations:
Guidance for Management of ARF in COVID-19
- World Health Organization (WHO) guidelines were revised 13 March, 20205. The guidelines call for High-flow nasal oxygen (HFNO) or NIV only to be used in selected patients with hypoxemic respiratory failure. The risk of treatment failure is high in patients with MERS treated with NIV, and patients treated with either HFNO or NIV should be closely monitored for clinical deterioration. The use of High-Flow nasal oxygen (which includes HVNI and HFNC) can proceed with caution. The therapy is reported to reduce the need for intubation compared to standard oxygen therapy. They call for the close monitoring of patients on the therapy, and specifically call for a one-hour trial on the therapy to determine if therapy needs to be discontinued. For both NIV and High Flow therapy the WHO points out the systems require good interface fitting to maintain a low risk of airborne transmission.
- The journal for the Society of Critical Care Medicine (Critical Care Medicine), has posted guidelines jointly with the Intensive Care Medicine journal, providing guidance for the use of High Flow Nasal Cannula. [17] The guidance calls for the use of HFNC in management of Acute Hypoxemic Respiratory Failure when conventional oxygen therapy fails, and that the use of HFNC was recommended over the use of NiPPV for these patients. NiPPV was only recommended if there were now HFNC available, and if the patient was not in urgent need of intubation. The authors also strongly recommended close monitoring of all patients receiving any non-invasive therapies, in case escalation to intubation and mechanical ventilation was required.
- Italian Thoracic Society/Associazione Italiana Pneumologi Ospedalieri calls for High Flow oxygen setup on all critical patients (part of necessary equipment is a gas delivery system to provide high flow oxygen gas). FiO2 of up to 0.9-1.0 to maintain saturation is called for. High Flow oxygen can be used either as a ‘ceiling option’ or a precursor to CPAP/NIV. Care must be taken due to possible droplet formation. When using CPAP/NIV, first choice is helmet interface without humidification, up to 15-20 cmH2 CPAP with a mask is second choice and NIV with a mask/filter is third choice.
- The Respiratory Care Committee of the Chinese Thoracic Society provide an expert consensus paper on preventing nosocomial transmission during respiratory care for critically ill patients with COVID-19 [18]. The recommendations are taken from Chinese experience, and the report states that, at writing, 1700 bedside clinicians have been infected. They acknowledge that respiratory treatment of the patients includes high-risk factors for nosocomial transmission. They call for specific interventions to mitigate the risk. Recommendations include use of Personal Protective Equipment (PPE), filters for ventilators and bag-valve-mask resuscitators, and masks for bronchoscopy. The guidelines also specifically call for use of a simple surgical mask over the face of the patient, covering the mouth and nose with the High Flow cannula in place, as well as recommendations to secure HFNC system tubing if it has the capability to disengage at the nosepiece (Vapotherm HVNI therapy uses a fused single-piece cannula without the possibility to thus disengage). They acknowledge that some technologies have heavy tubing sets and call for securing the tubing so as not to disturb the surgical mask.
- German Society for Internal Intensive Care Medicine and Emergency Medicine, The German Interdisciplinary Association for Intensive Care and Emergency Medicine, The German Society for Pulmonology and Breathing Medicine, The German Society for Anesthesiology and Intensive Care, and the ARDS Network of Germany issued guidelines on the intensive care therapies for patients with COVID-19 [19]. This interdisciplinary guidance calls for the use of both NIV and High Flow oxygen to maintain SpO2≥90%. They recognize that both modalities can lead to aerosol generation but cite Hui4 supporting safe administration assuming appropriate fit of the mask (NiPPV). The authors call for use of proper PPE and FFP2. The guidelines caution that for severe hypoxaemia (PaO2/FiO2 ≤ 200 mmHg) intubation / mechanical ventilation may be preferable, due to the increased risk of aerosol exposure during emergency intubation.
- A management paradigm was proposed by a French team, calling first for PPE and transmission control. [20] Possible admissions to the ICU should be considered on a daily basis. Care to COVID-19 patients should not be limited, but procedures which are likely to pose a transmission risk (e.g., ECMO, BAL, transport) must be discussed. ARDS is often associated with shock and multiple organ failure. HFNC is specifically called for in the treatment example, between standard oxygen cannula and mechanical ventilation. The example shows HFNC being applied while patient have been classified as having ARDS.
Known / Suspected Advantages and Disadvantages for HVNI
Interface Simplicity (v NIPPV)
The interface of the HVNI is a simple nasal cannula, requiring no exotic affixation tools (e.g., headgear, chinstraps, etc.). The interface is simple to size. The clinician will select a cannula from a group of 9 sizes, with prong diameters sized to occlude less than 50% of the area of the nostril, and prong separation based on the facial features (wider or narrower). The prongs are made of a pliable medical plastic, with a molded junction at the cannula tubing/prong interface (so as to prevent tubing becoming dislodged from the nosepiece), designed for continuous wear for 7-days.
The measurement and affixation of the nasal cannula is performed by clinical staff (e.g., Respiratory Care, Nursing, Medical). In the case of a patient who is combative or in whom facial contact is regularly an issue, simple paper tape on the cheeks can hold the cannula in place. Other forms of non-invasive ventilation such as CPAP or NiPPV require a tight-fitting air-tight facemask interface (helmet CPAP is available outside the United States, with that interface secured by an air-tight seal at the neck, not the face). Masks can be uncomfortable and must provide adequate seal around the variable geometry of the facial structure. Up to one-third of patients refuse the mask interface. [21] The mask must be removed for the patient to speak, ingest water or food, or oral medications. It is not uncommon to alternate masks on a single patient to reduce discomfort and reduce the possibility of skin breakdown. Every such interaction subjects the clinician to nosocomial exposure risk. The cannula allows the application of therapy whilst permitting the patient the opportunity to eat/drink/talk. The comfortable cannula interface may also facilitate prone posturing which is a recommended part of therapy for patients with ARD’s. Early application of HFNC plus prone posture in patients with moderate ARDS and a baseline SpO2 >95% avoided intubation. [22]
Hygienic Water Management
The Vapotherm Precision Flow® methodology for producing heated humidified gas involves heat and humidity transfer across a membrane. Hollow fibers surrounded by warm water allow medical gases to incorporate water vapor (at temperature) as the gas passes through the hollow fiber. The water path for this fiber acts as a filter, with pore sizes of approximately 0.005 microns. Thus, the gas path to the patient remains separated from bulk heated water, or ‘hot pot’.
Modest Distending Pressures
The HVNI generates modest amounts of pressure. While not likely a principle component of the mechanisms of action, the effect is not without significance for ARDS patients. A limited amount of information exists that demonstrates that HFNC generates some expiratory pressure. Measurements among surgical patients receiving HFNC showed the production of positive pressures of 1.5-1.9 cmH2O at 30 L∙min-1, 2.2-2.6 cmH2O at 40 L∙min-1, and 3.1-3.3 cmH2O at 50 L∙min-1. These modest amounts of PEEP may be advantageous in management ARDS patients.
Aerosol Generation and HVNI
Aerosols are produced when an air current moves across the surface of a film of liquid; the greater the force of the air the smaller the particles that are produced. Aerosol generating procedures (AGPs) are defined as any medical and patient care procedure that results in the production of airborne particles (aerosols). AGPs can produce airborne particles which can remain suspended in the air, travel over a distance and may cause infection if they are inhaled / contacted. Therefore, AGPs create the potential for airborne transmission of infections that may otherwise only be transmissible by the droplet route. [23]
Airborne transmission includes the movement of microorganisms from person to person by way of aerosols, which can contain infectious pathogens. [24-26] Understanding pathogen transmission can help provide essential measures to reduce spread.26 In the case of COVID-19, aerosolized forms of the pathogen may increase the risk of transmission as it is primarily spread by droplet transmission, as evidenced by SARS cases. “Production of infectious respiratory particles is dependent on the type and frequency of respiratory activity, type and site of infection, and pathogen load.” [26]
General Aerosol Particle Size and Transmission
Aerosolized infectious disease transmission is classified into two groups: (1) droplet transmission defined as expelled particles that can settle quickly that can travel short distances (within 1m) from the source, and (2) aerosol transmission defined as expelled particles with smaller size distributions that can thereby travel farther through air flows.25,26 The WHO (from 2007) has employed a 5um threshold to delineate between airborne (≤5um) and droplet-based (>5um) transmission. [26]
Exhaled aerosol is derived from the respiratory fluid lining the respiratory tract of the lungs. Proposed sites of aerosol production in the airway, include bronchiole aerosol, bronchial aerosol, and laryngeal aerosol. [27] Aerosol generation in these sites may be produced during shear and vibrational forces that can destabilize the mucous-air surface, which at bronchial sites may be at the highest velocity of air flow in the respiratory tract due to lowest cross-sectional areas at 8th-N of airway branching. The turbulent and high velocity airflow at this site can provide expiratory velocities up to 50 m∙sec-1 during cough and sneeze.27 Coughing has been shown to have flows in range of 360-400 L∙min-1. [28]
Particle sizes of droplets or aerosolized infectious pathogen can directly have bearing on transmission distance. Other factors such as room ventilation, people walking nearby, environmental factors, and air flows of any variety can influence particle dispersion distance from the host. [24-26] [29] [30] Researchers have proposed the following droplet size definitions: large droplet for >60um, small droplet for ≤60um, and droplet nuclei for <10um. The small droplets may participate in short range transmission but are also more likely than large droplets to evaporate and become droplet nuclei thereby involving a greater potential for long range transmission. [31] There are various environmental and physical factors that can influence aerosol particle size upon exhalation. A recent study concluded “bringing ventilation to recommended levels had the same mitigating effect as a vaccination coverage of 50% to 60%.” [32]
Aerosol Generating Procedures (AGP) in Clinical Management
AGPs are commonplace within hospitalized patients and may increase the risk of transmission to HCWs. These procedures occur independent of forms of oxygenation or ventilation that a patient may receive. Most AGP’s are present when an airway or its functionality is altered in some manner, causing the release of particles within aerosols from the lining of the airway. A partial list of AGP’s includes: intubation, extubation and related procedures such as manual ventilation and open suctioning, bronchoscopy, surgery and post-mortem procedures involving high-speed devices, some dental procedures (such as high-speed drilling), non-invasive ventilation (NIV) such as NiPPV and CPAP, High-Frequency Oscillating Ventilation (HFOV), High Flow Oxygen (HFNO, HFNC, and HVNI), induction of sputum, and any procedure that induces coughing. [2] [33]
Limited clinical evidence exists on the topic of nosocomial infection spread coupled with HFNC treatment. The CDC and WHO both recommend caution during the use of AGPs. HFNC has not been clinically shown to provide a significant risk of exhaled particle dispersion distance beyond simple nasal cannula oxygen. [34-36] In an analysis of SARS cases some specific AGPs were shown to have significant risk of transmission, but HFNC was clarified as not being a factor associated with significant transmission risk from patients to HCWs. [2] Recent modeling of exhaled air dispersion have shown that HFNC has no additional infection risk beyond standard oxygen facemasks, [34] exhaled air particle dispersion distance is shorter in HFNC than CPAP, [4] NiPPV facemasks/helmets provide a risk when not appropriately interfaced with skin/face, [1] and NiPPV may possibly have a higher risk of aerosol transmission in acute wards than when using oxygen by nasal cannula. [37]
When appropriately fitted interfaces are in place for NIV and HFNC, then HFNC dispersion distance is lower than found in NiPPV and CPAP. [3] [4] Fitting of face-mask for NiPPV/CPAP requires securing the mask margins to maintain an air-tight seal, tubing management, and affixation headgear. HVNI application is simplified to selection and placement of the nasal cannula in the nostrils without requirement for any seal. Also, Vapotherm HVNI devices use a fused cannula/tubing construction at the nosepiece, as opposed to some HFNC which can separate at the junction of the tubing and nosepiece (creating an airflow under the nose which is not directed into the patient). Clinicians must always wear Personal Protective Equipment (PPE) during patient interactions with suspected or confirmed COVID-19, but the presence of HFNC as a treatment modality should not present significant additional risk whilst providing appropriate clinical management for respiratory distress or hypoxemia. [35] [36] [38] WHO guidelines on COVID-19 also support these comments by stating “…that newer HFNC and NIV systems with good interface fitting do not create widespread dispersion of exhaled air and therefore should be associated with low risk of airborne transmission.” [36] In a mannequin model of liquid and bacterial pathogen dispersal, HFNC limited the dispersion to the area proximate to the face and cannula, suggesting that HFNC does not increase the risk of droplet and contact infection. [39]
According to latest CDC guidance, specific procedures performed on patients with known or suspected COVID-19 that may induce coughing (for example: sputum induction, open suctioning of airways, nebulization of medications) should be performed cautiously, and if possible avoided. A limited number of HCWs should be in the room if and when such procedures need to be performed. HCWs should wear PPE meeting current standards for inhalation, eye protection, and contact protection and ideally care would take place in Airborne Infection Isolation Rooms (AIIR) with environmental infection control in place after the procedure is completed. [5]
Proposed mitigation
Personal Protective Equipment / Room Environment
HFNC, NIV, and other respiratory therapies are used, [40] [41] and will continue to be used in the treatment of COVID-19 cases due to the need to address patient symptoms and balance resource availability. All respiratory therapy is, to some degree, an AGP, and aerosolized infectious droplets/particles are present in every patient’s normal breathing, talking, coughing, and sneezing. However, not all AGPs are associated with a known increased risk of transmission to HCWs. [2] PPE and environmental protection are cornerstones of risk mitigation in management of these patients.
All recommendations and suggested standards rely first on both environmental considerations (including room engineering) and the reliable application of PPE by HCWs. [10] [18] [19] [33] [36] [42-45] There has been a large amount of data as referenced by the CDC including several in-person onsite observational studies that demonstrate that HCW’s do not consistently adhere to recommended practices. [42] Bed placement (minimum distances), room air exchange, presence of doors, negative pressure, and other engineering considerations must also be taken into account when planning the management of patients undergoing AGPs.
There has been a wide range of adherence to Universal Precautions (the practice of treating all human blood and body fluids as if known to be infectious for HIV, HBV or other bloodborne pathogens). Appropriate glove use has been shown to be from 15-82%, however, during blood gas collection and resuscitation respectively adherence is from 92-98%. Among the studies reviewed by the Centers for Disease Control (CDC) [46], self-perceived adherence to infection control practices and adherence to Universal Precautions is much higher than that documented in observational studies. Publication in this area also demonstrates that as physicians and nurses remain in practice for longer periods of time adherence to these Universal Precautions decline. Education interventions and videotaping are used with frequency in many instances to identify adherence to system problems as well as enhance communication and access to protective equipment that may have become unfamiliar.
Surgical Facemask Covering Face with HVNI Nasal Cannula in Place
Masks have been extensively studied for the role in preventing transmission of pathogenic particles. [47-56] Surgical masks prevent diseases transmitted through droplets, from the healthcare worker to the patient, or vice versa. [57] [58] Masks are engineered to protect the environment from the individual using the mask, but when looking at respirators they are functioning to protect the individual from the environment. [59] Two factors are to be considered when questioning ability of the face mask to perform face seal leakage and filter medium. [57] Surgical masks have not yet been evaluated for use with High Velocity Nasal Insufflation (HVNI).
The application of the surgical mask in conjunction with HFNC has been recommended by the Chinese Thoracic Society. There are two types of masks, one is a surgical mask or face mask, and the other is a respirator. Surgical masks are used for the prevention of droplet transfer that travel short distances through coughing or sneezing. [60] The American Society for Testing and Materials Standard specification for performance of materials used in medical face masks (ASTM) rates masks from a level of minimum performance to maximum, with levels 1,2, and 3 being the intermediate measurements. Level 1 per ASTM is a mask for procedures with low amounts of fluid, spray, and/or aerosols produced, level 2 for procedures with moderate to light amounts of fluid, spray, and/or aerosols produced, level 3 for procedures with heavy to moderate amounts of fluid, spray, and/or aerosols produced
Related to viral transmission, the effect of the choice of mask by healthcare workers may not be clear. Data backing the effectiveness of a surgical mask vs. N95 respirator is somewhat limited. In a study looking at the N95 respirator in comparison to the surgical mask during the 2008-2009 influenza season, it was suggested that surgical masks were noninferior to the respirator when looking at influenza. [61] Surgical masks have been suggested as risk-mitigation adjuncts for aerosol generating procedures involving the nose and mouth. [18]
Computational Fluid Dynamic (CFD) Modeling of Particle Behavior with HVNI Under a Surgical Mask
Description of models
Two specific models were evaluated for the purpose of a) determining the effect of a Type 1 surgical mask over the mouth and nose with cannula in place on the fundamental action of the HVNI therapy (deadspace flush), and b) evaluate a simulation of the fluid dynamic behavior of the effect of the surgical mask on particles which may be generated in the airway whilst receiving HVNI therapy.
Methodology
Background
High velocity flow from a patient’s mouth and nose, such as that of a cough or sneeze can spread particles containing infectious diseases. These particles can range in size from sub-micron to larger than 1 mm. Surgical masks are commonly used on patients to control the spread of particles. These function by filtering the flow that pass through them. The masks do not, however form a perfect seal. There is usually some leakage, especially in the areas on either side of the nose, where the negative curvature of the patient’s face can leave a gap if not adjusted properly. What is unknown is whether a surgical mask can mitigate the spread of particles from a patient receiving HVNI therapy.
Computational Fluid Dynamics (CFD) allows for the simulation of complex flow fields, tracking of particles through those fields, and the absorption of particles within a porous media. Evaluation of the use of a surgical mask with HVNI was performed in ANSYS Fluent CFD (ANSYS, Inc, Canonsburg, PA, USA) and SolidWorks CFD (Dassault Systemes SolidWorks Corp, Waltham, MA, USA). For comparison, studies were performed on control cases of a patient on HVNI without a surgical mask, on low flow oxygen therapy (6 L∙min-1 via Nasal Cannula) with and without a surgical mask, and with a simulated breathing patient without any therapy with and without a surgical mask. Summary of all cases studied is listed here (Table 1).
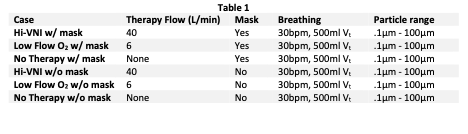
The use of a mask concurrently covering the face while receiving HVNI raises a concern that the trapping of CO2 inside the mask will reduce the effectiveness of the HVNI therapy. A SolidWorks CFD simulation has also been performed on a CT-derived anatomically accurate human airway model to compare flush of accessible extra-thoracic dead space, with and without a surgical mask.
Model Geometry, Assumptions, and Conditions
A 3D model of a human head is placed on a bed in a room, positioned 736mm above the floor with a 30° incline. The head model includes a simplified airway structure, a Vapotherm Adult Small / Pediatric Cannula and a surgical mask (Figure 1).

Room Model
The room measures 4.87m x 3.65m x 2.44m with a total volume of 43m3. Two 0.305m x 0.305m inlet vents are located on the ceiling and two 0.305m x 0.305m outlet vents on the wall opposite the patient. One of the outlet vents is located near the ceiling and one near the floor. A ventilation flow of 6 Air Changes per Hour (ACH) circulates the air in the room.
Mask, Cannula, and Head Model
A Type I surgical mask, appropriate for use on patients to prevent the spread of droplet particles carrying infectious diseases, is used for the simulation (Figure 2). The mask is fitted to a model of a human head. Since masks of this type are difficult to seal to the face in actual clinical practice, leaks were modeled in eight discreet locations (Figure 3). The total cross section of the leaks is 679mm2 (the case modeling ‘poor fit’ of the mask at the nose, in which the nose fit has not been formed to the face) which is equivalent in area to a 1.3mm gap all around the perimeter. One of the gaps simulates a cannula tube passing through the edge of the mask.


The mask properties were obtained from the standard governing surgical masks, EN14683 [62], and from Chan, et al., Aerosol penetration through surgical masks. [63] The mask is modelled as a porous medium which allows flow to pass through with a pre-defined resistance. The pressure drop of the mask is modelled to be in accordance with EN14683 and Chen, with a pressure drop of 29.4 Pa∙cm-2 with a test area of 4.9cm2. Particle penetration is obtained from Chen’s data for a mask with a filtration layer at 100L∙min-1. Since the flow rate through the mask does not exceed 80L∙min-1, this is conservative. Chen’s data does not include efficiency for particle sizes above 4µm, so the data was extrapolated with the assumption that for every additional 5µm, the percentage of particles passing through is halved. Filtration efficiency is shown below (Table 2). A User Defined Subroutine was created in ANSYS Fluent to remove particles that pass through the filter using the filtration efficiency.
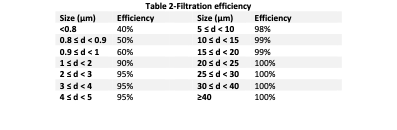
A Vapotherm® Adult Small / Pediatric cannula nose piece is positioned with its prongs in the nares. Flow of therapeutic gas is defined to be emitted from the prongs of the cannula.
Particle Model
The distribution of particle sizes emitted by a patient breathing, talking, coughing, and sneezing has been investigated in several studies. While the specific distributions vary, the range of particle sizes that are meaningful to study is generally from 0.1 to 100µm. Particles larger than 100µm are highly unlikely to penetrate a mask or travel far without a very high velocity flow. Particles smaller than 0.1µm account for a very small fraction of the total particles, are likely to escape regardless of a mask, and will remain airborne even in very low velocity fields.
The particle distribution (Table 3), for the simulation is taken from Exhaled droplets due to talking and coughing [30]. A Rosin-Rammler Diameter distribution method is used in the simulation to generate particles that approximate the particle distribution. Particles smaller than 0.1µm and larger than 100µm were not included. The Rosin-Rammler parameters, and n = 0.990 are used for the simulation. The curve fit for these parameters is shown (Chart 1).
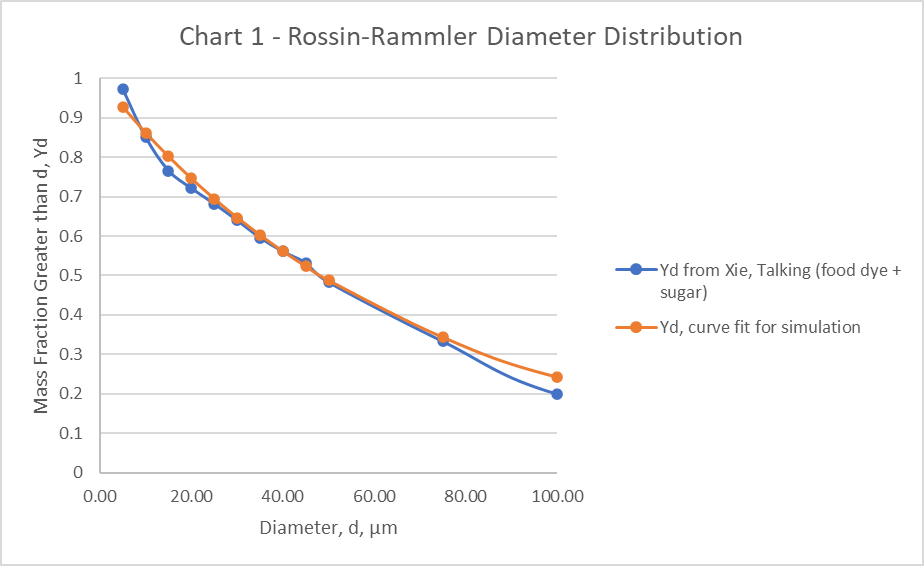

Mesh Geometry
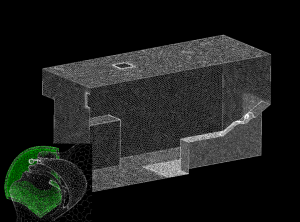
The mesh used in the simulation is tetrahedral with a total element count of 6 million converted to polyhedron elements with an element count of 1.1 million. Since the mask is crucial to the accuracy of the simulation, the mesh density within the mask is set to achieve a minimum of 4 elements through the thickness. Mesh refinement is also applied to the areas around the patient to achieve the best possible trajectories for particle movement near the patient and where particles are likely to settle. Mesh geometry used in the simulation is shown (Figure 4).
Flow Conditions
In all of the cases, the patient is modelled to be breathing at 32 breaths per minute with a tidal volume of 500mL. The breath curve is sinusoidal with a 1:1 inspiratory to expiratory ratio and no pause between the inspiratory and expiratory phases (i.e., no inter-breath interval). The cannula flow rate for HVNI therapy is 40 L∙min-1 and the flow rate for low-flow oxygen therapy is 6 L∙min-1.
Simulation

The simulations are transient, accounting for variation of the flow with time caused by cyclic breathing. The simulation is allowed to run until a simulated time of 5 hours has elapsed to allow the flow in the room to fully develop. Streamlines of the fully developed room ventilation flow are shown (Figure 5). Using a time step of 0.001sec, the simulations run for several breath cycles while particles are emitted into the flow. The particles are tracked through their trajectories and their final position is reported. The particles are grouped into one of the following categories:
- Caught in mask: This refers to particles that are absorbed in the filter and become trapped there. These particles are on a surface (the patient-worn mask) that would be considered contaminated in any case and only touched with protective measures. Thus, the particles in the mask are removed from consideration for ‘additional contact exposure risk’ and are dealt with as ‘biohazard’.
- Trapped in vicinity of patient: Particles that are outside the mask, and are deposited on the patient’s head, upper torso, bead and pillow, with a path length of less than 1m. These particles are deposited in an area that would normally be considered likely risk, and only be touched with protective equipment, but a caregiver in close proximity to the patient could intercept airborne particles, thus reinforcing the need for PPE and environmental awareness.
- Escaped: Particles that have travelled more than 1m and remain airborne for a longer time, exit through the ventilation outlet, or are deposited on another surface within the room. These particles may deposit on any surface in the room or be inhaled be anyone in the room prior to exiting through ventilation. These particles are of particular note when attempting to reduce transmission risk.
CO2 Flush Simulation:

A CFD simulation was performed with the following inputs: 24 breath per minute, 500mL tidal volume, 35 L∙min-1 through a Vapotherm Adult Small / Pediatric Cannula. The patient was modelled to exhale 8% CO2 by mass. The model uses an anatomically competent complete human head model derived from CT-scan of a petite adult female with accurate rendering of the airway space, and a model surgical mask in place over the nose and mouth (Figure 6). Figure 6 shows the model used for this simulation. The flow resistance of the modeled mask is based on the properties defined in EN14683. Flush is measured by simulating a complete breath cycle without therapy and totalizing the CO2 that is re-inhaled during the subsequent inspiratory phase. The simulation is also run on the models with therapy, again totalizing the amount of CO2 that is inhaled during the inspiratory phase. The reduction in the amount of CO2 is then calculated by comparing the therapy simulations to the baseline simulation without therapy.
Results:
Velocity: One of the key factors affecting dispersion of particles is the velocity of the flow that contains the particles. Larger particles may remain suspended longer in a flow which has a higher velocity. The simulations show that in all cases the velocity of the expiratory flow is substantially reduced by the presence of a mask. The mask resists high velocity flow through the media and distributes the flow through a larger area. This diffusing effect results in a much lower velocity in the area near the patient’s face and does not allow jetting of flow over long distances. Figures 7 through 12 show the velocity field, along the midline plane, is shown for all cases.
Leakage: Some of the flow does not pass through the mask, but rather exits through the leaks. While this is not an insubstantial percentage of the flow at peak expiratory, ranging from 11.6% for the case without therapy to 16.5% for Hi-VNI therapy, the velocity is reduced similarly to the flow that passes through the mask. Figures 13 through 18 show isosurfaces for all cases at peak expiratory flow. All points on the surfaces have a velocity of 0.5 m∙sec-1. In the cases where a mask is present, the velocity is both reduced and is redirected back toward the patient rather than out into the room. Table 4 shows the leakage percentage for each case with a mask at peak expiratory.
Particle dispersion: With a mask in place, most of the flow passes through the mask and is filtered to remove approximately 96.5% of particles by mass. The flow that does escape through the leaks has followed a tortuous path which tends to cause the larger particles to impact the surface of the mask, face and cannula. These particles have a path length of less than 1m and remain trapped in the areas around the patient’s head.
Figures 19 through 24 show the particle trajectories for all cases at peak inspiratory flow. Each path represents 0.1% of the total mass of particles. Trajectory color indicates the particle size in µm, with red indicating large particles, yellow indicating medium particles and blue indicating the smallest particles. In all cases with a mask in place, there are no particle trajectories remaining for the larger particles (red, green, and yellow trajectories).
Table 5 shows the percentage of mass that has accumulated in the three regions of interest. With HVNI at 40 L∙min-1 and the addition of a mask, 83.2% of the particle mass is captured and terminated in the mask. That is compared to 73.6% of the particle mass captured in the mask whilst on Low Flow Oxygen at 6 L∙min-1, and 87.2% for tidal breathing with a mask. It is important to note that the proportion of droplets (i.e., ≥ 5μm) which are captured in the mask over HVNI therapy is 85.9%, as compared to 75.9% while receiving Low Flow oxygen at 6 L∙min-1, and 89.9% performing tidal breathing. It is also important to note that the particles which escape the mask during HVNI have a longer travel length, with 15.9% of particles travel greater than 1m. That is compared to 6.9% on Low Flow oxygen at L∙min-1 (which has fully 19.5% of particle mass lands and is trapped at <1m from the mask). Of note, if the patient is tidal breathing in the room, without therapy and without a surgical mask, 31% of particle mass leaving the nose and mouth will deposit greater than one meter from the face.



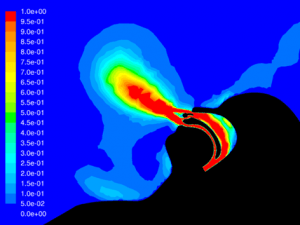



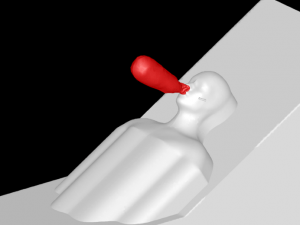


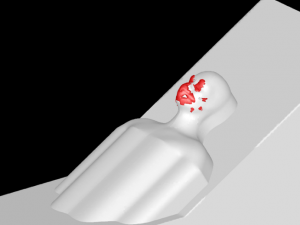
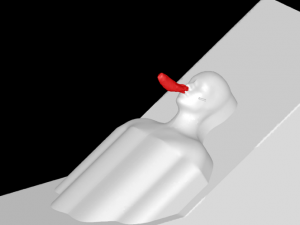

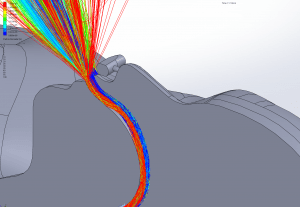
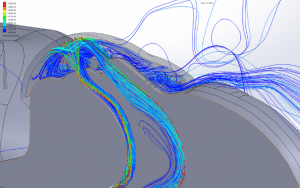



CO2 Flush:
With a Type I Surgical mask, significant CO2 is still flushed from the airway using Hi-VNI therapy. Presence of the mask did, however, reduce flush from 62.1% to 38.9%. This is due to some trapping of CO2 in the mask. Figure 25 shows remaining CO2 at end-expiratory with a mask and Figure 26 shows remaining CO2 without a mask. Increasing the flow rate from 35 L∙min-1 to 40 L∙min-1 with the mask in place improved flush to 52.0% but did not completely compensate for the mask.


Summary of CFD Findings
Hi-VNI therapy, like all respiratory therapies generates particles that may become airborne and spread over a large area. A surgical mask placed over the mouth and nose of the patient significantly reduces the spread of these particles by reducing the flow velocity and trapping particles. Using HVNI with a mask in place, the percentage of particles escaping to the mask is less than that of a patient who is tidal breathing without therapy in the room and is less than the amount escaping the mask during low flow oxygen at 6 L∙min-1 wearing a surgical mask. In all cases, lower flow and the presence of a mask reduced particle dispersion. An important finding was that the overwhelming majority of particles escaping the mask were associated with the leaks built into the simulation model. While these are intended to simulate a ‘worst case’ real world clinical application, these findings suggest attention must be paid to securing the mask to the face.
Further, the mask captured a greater proportion of droplet (≥ 5μm) sized particles than were captured while receiving Low Flow oxygen at 6 L∙min-1. The WHO has suggested the primary mode of transmission was droplet.8 This has been brought into some question with more recent findings suggesting the maintenance of viral activity in smaller (aerosol) particles. [64] These findings support the notion that a mask over the nasal interface during HVNI can substantially reduce the particulate burden in the room around the patient.
Conclusion
- All respiratory care represents a risk of AGP during the care of patients with COVID-19.
- Personal Protective Equipment and Environmental Control/Engineering should be the initial concern and consideration when managing patients with COVID-19.
- High Velocity Nasal Insufflation therapy is established as a competent means of supporting both oxygenation and ventilation for patients with acute respiratory distress, including pneumonia.
- The use of HVNI as a form of High Flow Oxygen delivery is unlikely to represent a disproportionate risk of droplet exposure as compared to other forms of ventilatory support and may have an advantage over other NIV administration.
- The use of HVNI along with a surgical facemask in place over the cannula has been shown in modeling to not remove the fundamental therapeutic characteristic of extra-thoracic deadspace flush.
- The administration of HVNI along with a surgical facemask in place over the cannula has been shown in modeling of an adult receiving HVNI at 40 L∙min-1 to have the percent particles either airborne or depositing on a surface beyond the immediate area of the patient to be no different and show no greater dispersal of particles than low flow oxygen or tidal breathing with a facemask in place. Capture in the mask is was less than that captured by the mask during low oxygen therapy, and less than a patient performing tidal breathing without a mask.
REFERENCES
[1] Cheung JC, Ho LT, Cheng JV, Cham EYK, Lam KN. Staff safety during emergency airway management for COVID-19 in Hong Kong. Lancet Respir Med. 2020.
[2] Tran K, Cimon K, Severn M, Pessoa-Silva CL, Conly J. Aerosol generating procedures and risk of transmission of acute respiratory infections to healthcare workers: a systematic review. PLoS One. 2012;7(4):e35797.
[3] Hui DS, Chow BK, Lo T, et al. Exhaled air dispersion during noninvasive ventilation via helmets and a total facemask. Chest. 2015;147(5):1336-1343.
[4] Hui DS, Chow BK, Lo T, et al. Exhaled air dispersion during high-flow nasal cannula therapy versus CPAP via different masks. The European respiratory journal. 2019;53(4).
[5] Organization WH. Clinical management of severe acute respiratory infection when Novel coronavirus (2019-nCoV) infection is suspected: Interim Guidance. WHO reference number: WHO/nCoV/Clinical/2020.3 13 March 2020 2020.
[6] Zhou P, Yang XL, Wang XG, et al. A pneumonia outbreak associated with a new coronavirus of probable bat origin. Nature. 2020;579(7798):270-273.
[7] Wu Z, McGoogan JM. Characteristics of and Important Lessons From the Coronavirus Disease 2019 (COVID-19) Outbreak in China: Summary of a Report of 72314 Cases From the Chinese Center for Disease Control and Prevention. JAMA. 2020.
[8] WHO. Report of the WHO-China Joint Mission on Coronavirus Disease 2019 (COVID-19). 2020.
[9] Center for Systems Science and Engineering Johns Hopkins Univeristy. Coronavirus COVID-19 Global Cases. 2020; https://gisanddata.maps.arcgis.com/apps/opsdashboard/index.html#/bda7594740fd40299423467b48e9ecf6.
[10] Grasselli G, Pesenti A, Cecconi M. Critical Care Utilization for the COVID-19 Outbreak in Lombardy, Italy: Early Experience and Forecast During an Emergency Response. JAMA. 2020.
[11] Atwood CW, Camhi S, Little KC, et al. Impact of heated humidified high flow air via nasal cannula on respiratory effort in patients with chronic obstructive pulmonary disease. Chronic Obstructive Pulmonary Disease. 2017;4(4):279-286.
[12] Miller TL, Saberi B, Saberi S. Computational Fluid Dynamics Modeling of Extrathoracic Airway Flush: Evaluation of High Flow Nasal Cannula Design Elements. Journal of Pulmonary & Respiratory Medicine. 2016;6(5):376.
[13] Dysart K, Miller TL, Wolfson MR, Shaffer TH. Research in high flow therapy: mechanisms of action. Respiratory medicine. 2009;103(10):1400-1405.
[14] Doshi P, Whittle JS, Bublewicz M, et al. High-Velocity Nasal Insufflation in the Treatment of Respiratory Failure: A Randomized Clinical Trial. Ann Emerg Med. 2018;72(1):73-83 e75.
[15] Frat JP, Thille AW, Mercat A, et al. High-flow oxygen through nasal cannula in acute hypoxemic respiratory failure. The New England journal of medicine. 2015;372(23):2185-2196.
[16] Ni YN, Luo J, Yu H, et al. Can High-flow Nasal Cannula Reduce the Rate of Endotracheal Intubation in Adult Patients With Acute Respiratory Failure Compared With Conventional Oxygen Therapy and Noninvasive Positive Pressure Ventilation?: A Systematic Review and Meta-analysis. Chest. 2017;151(4):764-775.
[17] Alhazzani W, Moller MH, Arabi YM, et al. Surviving Sepsis Campaign: Guidelines on the Management of Critically Ill Adults with Coronavirus Disease 2019 (COVID-19). Critical care medicine. 2020;PREPUBLICATION.
[18] Respiratory care committee of Chinese Thoracic S. [Expert consensus on preventing nosocomial transmission during respiratory care for critically ill patients infected by 2019 novel coronavirus pneumonia]. Zhonghua jie he he hu xi za zhi = Zhonghua jiehe he huxi zazhi = Chinese journal of tuberculosis and respiratory diseases. 2020;17(0):E020.
[19] Kluge S, Janssens U, Welte T, Weber-Carstens S, Marx G, Karagiannidis C. [Recommendations for critically ill patients with COVID-19]. Medizinische Klinik, Intensivmedizin und Notfallmedizin. 2020.
[20] Bouadma L, Lescure FX, Lucet JC, Yazdanpanah Y, Timsit JF. Severe SARS-CoV-2 infections: practical considerations and management strategy for intensivists. Intensive care medicine. 2020.
[21] Carron M, Fero U, BaHammam AS, et al. Complications of non-invasive ventilation techniques: a comprehensive qualitative review of randomized trials. British journal of anaesthesia. 2013;110(6):896-914.
[22] Ding L, Wang L, Ma W, He H. Efficacy and safety of early prone positioning combined with HFNC or NIV in moderate to severe ARDS: a multi-center prospective cohort study. Critical care. 2020;24(1):28.
[23] WHO. Infection prevention and control of epidemic and pandemic prone acute respiratory infections in health care. Geneva, Switzerland 2014.
[24] Tang JW, Li Y, Eames I, Chan PK, Ridgway GL. Factors involved in the aerosol transmission of infection and control of ventilation in healthcare premises. J Hosp Infect. 2006;64(2):100-114.
[25] Kwon SB, Park J, Jang J, et al. Study on the initial velocity distribution of exhaled air from coughing and speaking. Chemosphere. 2012;87(11):1260-1264.
[26] Gralton J, Tovey E, McLaws ML, Rawlinson WD. The role of particle size in aerosolised pathogen transmission: a review. J Infect. 2011;62(1):1-13.
[27] Patterson B, Wood R. Is cough really necessary for TB transmission? Tuberculosis (Edinb). 2019;117:31-35.
[28] Mellies U, Goebel C. Optimum insufflation capacity and peak cough flow in neuromuscular disorders. Ann Am Thorac Soc. 2014;11(10):1560-1568.
[29] Yang S, Lee GW, Chen CM, Wu CC, Yu KP. The size and concentration of droplets generated by coughing in human subjects. J Aerosol Med. 2007;20(4):484-494.
[30] Xie X, Li Y, Sun H, Liu L. Exhaled droplets due to talking and coughing. J R Soc Interface. 2009;6 Suppl 6:S703-714.
[31] Xie X, Li Y, Chwang AT, Ho PL, Seto WH. How far droplets can move in indoor environments–revisiting the Wells evaporation-falling curve. Indoor Air. 2007;17(3):211-225.
[32] Smieszek T, Lazzari G, Salathe M. Assessing the Dynamics and Control of Droplet- and Aerosol-Transmitted Influenza Using an Indoor Positioning System. Sci Rep. 2019;9(1):2185.
[33] England DoHaSC-PHW-PHANI-HPS-PH. Guidance for infection prevention and control in healthcare settings: Adapted from Pandemic Influenza Guidance for Infectoin Prevention and Control in Healthcare Settings 2020. 2020.
[34] Leung CCH, Joynt GM, Gomersall CD, et al. Comparison of high-flow nasal cannula versus oxygen face mask for environmental bacterial contamination in critically ill pneumonia patients: a randomized controlled crossover trial. J Hosp Infect. 2019;101(1):84-87.
[35] CDC. Interim Clinical Guidance for Management of Patients with Confirmed Coronavirus Disease (COVID-19). 2020, March 7, 2020.
[36] WHO. Clinical management of severe acute respiratory infection when Novel coronavirus (2019-nCoV) infection is suspected: Interim Guidance. WHO/nCoV/Clinical/2020.3 28 January 2020 2020.
[37] Wong BC, Lee N, Li Y, et al. Possible role of aerosol transmission in a hospital outbreak of influenza. Clin Infect Dis. 2010;51(10):1176-1183.
[38] Chang, Xu H, Rebaza A, Sharma L, Dela Cruz CS. Protecting health-care workers from subclinical coronavirus infection. Lancet Respir Med. 2020;8(3):e13.
[39] Kotoda M, Hishiyama S, Mitsui K, et al. Assessment of the potential for pathogen dispersal during high-flow nasal therapy. J Hosp Infect. 2019.
[40] Xu Z, Shi L, Wang Y, et al. Pathological findings of COVID-19 associated with acute respiratory distress syndrome. Lancet Respir Med. 2020.
[41] Auwaerter PG. Coronavirus COVID-19 (SARS-2-CoV). Johns Hopkins ABX Guide March 8, 2020.
[42] CDC. Infection Control: II Fundamental Elements Needed to Prevent Transmission of Infectious Agents in Healthcare Settings. 2007; https://www.cdc.gov/infectioncontrol/guidelines/isolation/prevention.html. Accessed 17 March 2020, 2020.
[43] WHO. Clinical management of severe acute respiratory infection when novel coronavirus (2019-nCoV) infection is suspected (13 March 2020). 2020.
[44] Murthy S, Gomersall CD, Fowler RA. Care for Critically Ill Patients With COVID-19. JAMA. 2020.
[45] Harari SA, Vitacca M, Blasi F, Centanni S, Santus PA, Tarsia P. Managing the Respiratory care of patients with COVID-19. http://www.aiponet.it: Italian Thoracic Society – Associazione Italiana Pneumologi Ospedalieri – Societa Italiana Di Pneumologia;2020.
[46] CDC. Interim Infection Prevention and Control Recommendations for Patients with Suspected or Confirmed Coronavirus Disease 2019 (COVID-19) in Healthcare Settings. 2020.
[47] Ricard JD. High flow nasal oxygen in acute respiratory failure. Minerva anestesiologica. 2012;78(7):836-841.
[48] Lenglet H, Sztrymf B, Leroy C, Brun P, Dreyfuss D, Ricard JD. Humidified high flow nasal oxygen during respiratory failure in the emergency department: feasibility and efficacy. Respiratory care. 2012;57(11):1873-1878.
[49] Yin M, Shen H. [Does the humidified high-flow nasal oxygen therapy decrease need for intubation in the acute respiratory failure adult patient?]. Zhongguo wei zhong bing ji jiu yi xue = Chinese critical care medicine = Zhongguo weizhongbing jijiuyixue. 2012;24(2):68-69.
[50] Quesnel LB, 1975, The efficiency of surgical masks of varying design and composition, Br J Surg.; 62 (12):936-40.
[51] Barbosa MH and Graziano KU, 2006, Influence of wearing time on efficacy of disposable surgical masks as microbial barrier, Brazilian Journal of Microbiology 37:216-217.
[52] Braunlich J, Beyer D, Mai D, Hammerschmidt S, Seyfarth HJ, Wirtz H. Effects of nasal high flow on ventilation in volunteers, COPD and idiopathic pulmonary fibrosis patients. Respiration; international review of thoracic diseases. 2013;85(4):319-325.
[53] European Standard EN 149 + A1, 2009, Respiratory protective devices – Filtering half masks to protect against particles – Requirements, testing, marking.
[54] ASTM International Standard F1862, 2007, Standard test method for resistance of medical facemasks to penetration by synthetic blood (horizontal projection of fixed volume at a known velocity).
[55] prEN European Standard 14683, 2012, CEN/TC 205/WG14/PG1, Medical facemasks – Requirements and test methods.
[56] Bowen, L. E. (2010). Does That Face Mask Really Protect You? Applied Biosafety, 15(2), 67–71. .
[57] Roca O, Riera J, Torres F, Masclans JR. High-flow oxygen therapy in acute respiratory failure. Respiratory care. 2010;55(4):408-413.
[58] Hinds, W. C. (1999). Aerosol Technology: properties, behavior, and measurement of airborne particles. 2nd Edition. New York: Wiley –Interscience.
[59] Balazy A, Toivola M, Adhikari A, Sivasubramani SK, Reponen T, Grinshpun SA. Do N95 respirators provide 95% protection level against airborne viruses, and how adequate are surgical masks? Am J Infect Control. 2006;34(2):51-57.
[60] Sanchez, Erin, “Filtration Efficiency of Surgical Masks” (2010). Graduate Theses and Dissertations.
[61] Loeb M, Dafoe N, Mahony J, et al. Surgical Mask vs N95 Respirator for Preventing Influenza Among Health Care Workers: A Randomized Trial. JAMA. 2009;302(17):1865–1871. doi:10.1001/jama.2009.1466.
[62] Standard E. Surgical Masks – Requirements and Test Methods. 2005.
[63] Chen C-C, Willeke K. Aerosol penetration through surgical masks. American Journal of Infection Control. 1992;20(4):177-184.
[64] van Doremalen N, Bushmaker T, Morris DH, et al. Aerosol and Surface Stability of SARS-CoV-2 as Compared with SARS-CoV-1. The New England journal of medicine. 2020.